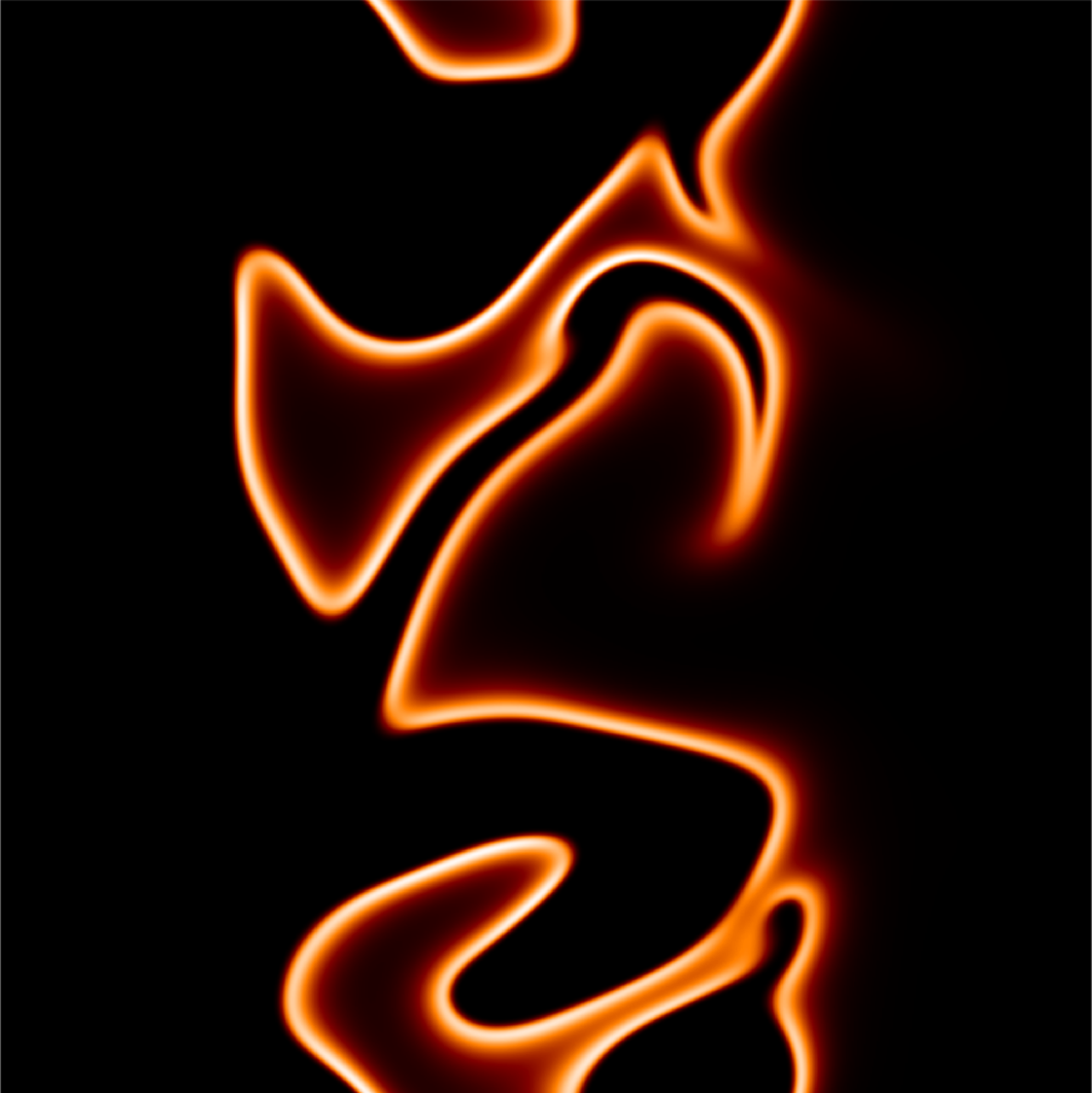
SCHEDULE
1:30 pm
Welcome and introduction
2:00pm - 5:30pm
Challenges in multi-scale modelling, methods and applications.
Prof. Rodney Fox
9:00am - 12:30pm
Detailed kinetic mechanisms for reacting flows: uncertainty quantification, validation and reduction.
Prof. Tamas Turanyi
12:30pm - 2:00pm
Lunch
2:00pm - 5:30pm
Multi-scale methods for fluidized bed reactors.
Prof. Hans Kuipers
9:00am - 12:30pm
Lattice Boltzmann simulations of single-phase and two-phase flow systems.
Prof. Harry Van den Akker
12:30pm - 2:00pm
Lunch
2:00pm - 5:30pm
Quadrature-Based Moment Methods for Multiphase Chemically Reacting Flows
Prof. Rodney Fox
MONDAY, JAN 23rd
TUESDAY, JAN 24th
WEDNESDAY, JAN 25th
9:00am - 12:30pm
Collaboration of experiments and simulations for the development of predictive models: application to full-scale boilers.
Prof. Philip J. Smith
1:00 pm
Farewell
FRIDAY, JAN 27th
9:00am - 12:30pm
Soot modeling, methods and applications.
Prof. Heinz Pitsch
12:30pm - 2:00pm
Lunch
2:00pm - 5:30pm
Moment methods for the description of polydisperse sprays or aerosols.
Dr. Frédérique Laurent-Nègre
THURSDAY, JAN 26th
About the speakers and the contributions
Prof. Rodney Fox
Professor Fox joined Iowa State University as the Glenn Murphy Professor of Engineering in 1999, and was the Herbert L. Stiles Professor of Chemical Engineering from 2003-2012. He was promoted to Distinguished Professor in Engineering in 2010. Prior to joining ISU, Fox was an Associate Professor of Engineering at Kansas State University, and has held visiting professorships in Denmark, France, Italy, Switzerland and the Netherlands. From 1987-88, he was a NATO Postdoctoral Fellow at LSGC in Nancy, France working in the area of chemical reaction engineering under the guidance of Prof. Jacques Villermaux. His numerous professional awards include a NSF Presidential Young Investigator Award in 1992 and the ISU Outstanding Achievement in Research Award in 2007. Professor Fox was elected Fellow of the American Physical Society in 2007. From 2012-14, he was a Marie-Curie Senior Fellow at the Ecole Centrale in Paris, France. He is currently an Associate Scientist at the US-DOE Ames Laboratory.
Abstract: Computational fluid dynamics (CFD) was originally developed to investigate single-phase fluid flows for relatively simple technological and environmental applications. Over the past 30+ years, the range of applications has greatly expanded to include turbulent, multiphase, reacting, and other complex flows. As a result, the mathematical models used for CFD have become much more sophisticated to accommodate a wider range of physical phenomena. The development of these models is based on physical/chemical processes occurring at length and time scales that cannot be resolved in a CFD simulation. For this reason, multiscale modeling plays a critical role in modern CFD codes. For example, molecular-scale processes determine chemical reaction rates, nanoscale processes govern aggregation of colloids and fine particles, and microscale processes generate clusters in particle-laden flows. In CFD simulations of cutting-edge technologies, these multiscale processes combined with the unresolved flow scales due to turbulence, must be properly accounted for in order to achieve accurate predictions. Specific examples of multiscale CFD models will be used to illustrate the current state of the art.
Prof. Hans Kuipers
graduated in 1985 at the department of Chemical Engineering of the University of Twente. In December of the same year he started with his Ph.D. study at the Reaction Engineering group of University of Twente on detailed micro balance modeling of gas-fluidized beds. In June 1990 he received his Ph.D. degree in Chemical Engineering and was appointed as assistant professor in the Reaction Engineering group headed by Prof. W.P.M. van Swaaij. In 1994 he was appointed as associate professor in the same group. In 1999 he became fulltime professor in Fundamentals of Chemical Reaction Engineering at the University of Twente. Since August 2010 he is a fulltime professor at Eindhoven University of Technology and heads the group Multiphase Reactors. His research interests are in the area of multiphase reactors.
Abstract: Dense multiphase flows involving momentum, mass and heat transfer are frequently encountered in industrial processes involving granulation, coating and polymerization. In these flows the complex phase interactions govern the prevailing flow phenomena such as the formation and evolution of heterogeneous structures to a large extent. These structures have significant impact on the quality of the contact between the phases and as a direct consequence thereof strongly affect reactor performance. Additional complexities arise due to enhanced dissipation due to wet particle-particle collisions which prevail in processes such as spray drying, coating, granulation and gas phase polymerization.
Due to the inherent complexity of these multiphase flows a multi-scale modeling approach is adopted in which the interactions between the phases can be properly accounted for. The idea is essentially that detailed models are used to generate closures for the interphase transfer coefficients to feed continuum models which can be used to compute the system behavior on a much larger (industrial) scale. In this contribution recent advances in the area of multi-scale simulation of dense multi-phase flows will be highlighted with emphasis on coupled mass, momentum and heat transfer. In addition, areas which need substantial further attention will be discussed.
Prof. Harry Van den Akker
is Bernal Professor of Fluid Mechanics at the University of Limerick from September 2013. Prior to joining academia, Professor Van den Akker was a research engineer at Shell Research in Amsterdam. He has held visiting appointments in Princeton and at King’s College London. He was President of the Dutch Physical Society for 6 years, and Scientific Director of the Netherlands Research School in Process Technology for 12 years. His current research interests are in developing lattice-Boltzmann techniques for describing meso-scale transport, transfer, and phase change processes in fluids and materials.
Prof. Tamas Turanyi
graduated as a chemist in 1983 at the Eötvös Loránd University (ELTE), Budapest, Hungary. He also received an MSc in Applied Mathematics in 1988 at the same university. He started to work in the field of experimental gas kinetics in the Central Research Institute for Chemistry of the Hungarian Academy of Sciences in Budapest. He received his PhD degree in 1988 at the ELTE and was a postdoc in the group of Prof Mike Pilling at the School of Chemistry, University of Leeds, UK, in years 1990-92 and 1994-95. He is working in the Institute of Chemistry of the Eötvös Loránd University since 1996. He became a full professor of chemistry in 2007. His main research interest is the simulation of chemical kinetic models based on detailed reaction mechanisms; development, analysis, uncertainty quantification and reduction of reaction mechanisms. The results are used in combustion, atmospheric chemistry and biochemical simulations.
Abstract: Detailed reaction mechanisms for combustion systems can be constructed using three main information sources: direct measurements of the rate coefficients of elementary reactions, high level theoretical calculations of the rate coefficients, and indirect measurements, like measurement of ignition delay time and laminar burning velocity. A systematic procedure was suggested that takes into account of uncertainty of the rate coefficients based on literature sources (called prior uncertainty), and assesses the uncertainty of the indirect experimental data. For a given combustion system, all published information is considered in these three categories, and the rate parameters of the model are fitted to the collected data. The result is an optimized combustion mechanism and a mathematical statistics-based assessment of the uncertainty of the model parameters (called posterior uncertainty). This posterior uncertainty is much narrower compared to the prior uncertainty for the fitted parameters. The obtained optimized mechanism is benchmarked against other similar mechanisms using the collected experimental data. Also, the obtained mechanism can be reduced to make it applicable in CFD calculations. The basic methods for mechanism reduction are briefly discussed.
Prof. Heinz Pitsch
received his PhD from RWTH Aachen University in 1998. He had two subsequent postdoctoral positions at UC San Diego and Stanford University and was from 2003 – 2013, Assistant and Associate Professor at Stanford University. Since 2010, he is Professor in Mechanical Engineering and director of the Institute for Combustion Technology at RWTH Aachen University. His main research interests are in the fields of combustion theory, combustion chemistry, turbulence, and multi-phase flows with application to technical combustion systems.
Abstract: Modeling combustion in practical combustion systems is challenging, especially when finite rate chemical kinetics and their interaction with turbulence become of leading order importance. This is typically the case for pollutants formation, which usually involves slow chemical processes. Predicting soot formation and oxidation is particularly complicated because of the complex interaction of the involved multi-physics aspects, which requires a large number of model components, such as chemical kinetic mechanisms, fuel surrogate formulations, a statistical description of soot particles, and turbulence closure models for the soot transport equations. In this course, new developments for all these aspects will be discussed and modeling challenges revealed. In addition, a priori and a posteriori validation studies, for instance, for different laboratory-scale turbulent flame experiments will be shown. Finally, applications of detailed soot models in large-eddy simulations are discussed for different examples including aircraft gas turbine engines and internal combustion engines.
Dr. Frédérique Laurent-Nègre
is a tenured research scientist of CNRS at EM2C Laboratory of CentraleSupélec and CNRS, member of the Fédération de Mathématiques. She is an expert in the field of mathematical modeling, numerical analysis and simulation of two-phase flows, with a special focus on moment methods for population balance equations for polydisperse sprays and soots. In the last ten years, she has built up, together with Marc Massot, an applied mathematics team in this engineering laboratory, fostering interdisciplinary research and innovative methods in her field of expertise, both in mathematics and for engineering applications in collaboration with research institutes such as IFPEn and ONERA, or with industry.
Abstract: Sprays and aerosols are present in many applications such as in internal combustion engines, where the injected fuel is atomized in a spray and soots are produced, or in solid rocket motors where alumina sprays comes from the combustion of aluminium particles added in the propellant. The size and eventually the velocity polydispersion of such populations of droplets or particles has then to be taken into account at a reasonable cost. For that purpose, moment methods represent an interesting strategy, eventually coupled with a discretization along the size variable.
The course will address the Mathematical basis of moment methods, the derivation of the moment equations from the (Generalized) Population Balance Equation, leading to a hierarchy of models and then, numerical schemes adapted to the mathematical properties of the obtained equations and allowing to preserve the realizability of the moments. Some examples of applications will also be shown.
Prof. Philip J. Smith
is professor of Chemical Engineering at the University of Utah Professor and director of the Institute for Clean and Secure Energy (ICSE). He is also the chair of the American Flame Research Committee (AFRC) of the International Flame Research Foundation. Until 2010, Prof. Smith has been responsible for the hydrocarbon fire simulation aspects of CSAFE, the Center for the Simulation of Accidental Fires and Explosions, one of five DOE funded Advanced Simulation and Computing (ASC) university alliance centers. A number of continuing projects built upon the C-SAFE legacy. These include The Carbon-Capture Multidisciplinary Simulation Center (CCMSC). CCMSC is a National Nuclear Security Administration (NNSA) multidisciplinary center of excellence in the emerging field of predictive science, funded by NNSA’s Predictive Science Academic Alliance Program II (PSAAP II).
Abstract: Predictions from computer simulations are increasingly being used to make decisions for society. The value of the computer simulation is proportional to the confidence placed in the uncertainty associated with the prediction. Multi-scale, multi-physics predictions are particularly susceptible to uncertainties in the model form used to represent the physical phenomena driving the predictive quantity of interest. These uncertainties can only by quantified through a formal evaluation of consistency between the simulations and the measurements. But, seldom can the measurements be made in the system where the prediction is sought; nor can the measurements be made with the degree of accuracy desired. While both of these constraints confound the uncertainty analysis; the predictivity objective will be most effectively realized when the model form follows the maxim Roger Sessions attributed to Albert Einstein: “Everything should be made as simple as possible, but not simpler." A methodology for performing top-down, hierarchical collaboration between experimental and simulation data to quantify predictivity in the presence of model form uncertainty will be shown through the example of a multi-phase, reacting-flow power boiler application.